UA Mechanical Engineer Builds Custom-Made Body Parts in the Lab
UA biomechanical research aims to improve medical device design and identify relationships between structure and function in soft tissues.
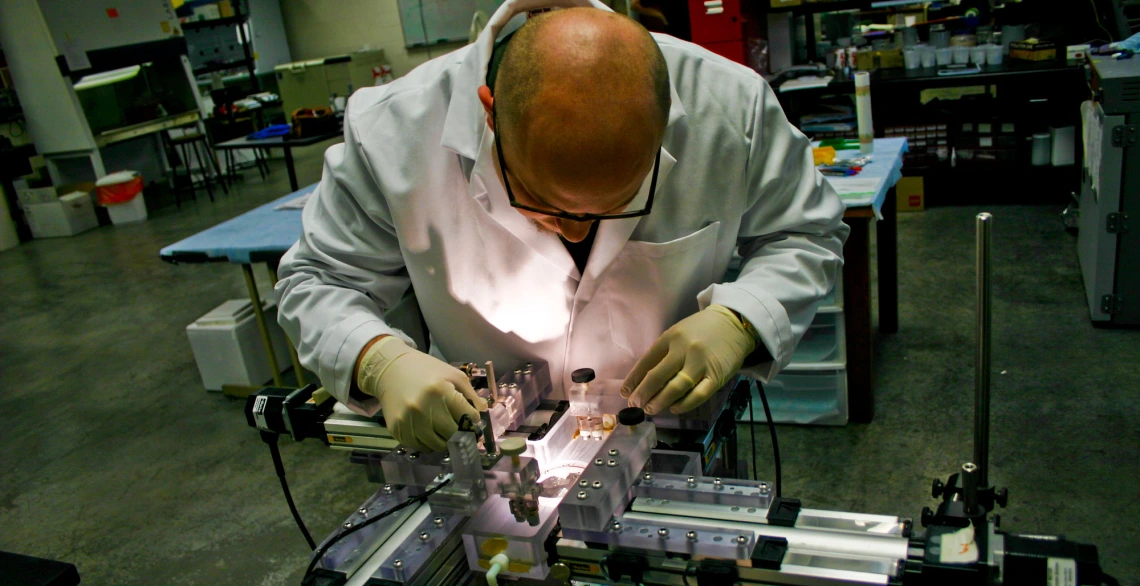
Assistant professor Jonathan Vande Geest prepares a sample for biomechanical testing in his lab.
Jonathan Vande Geest’s laboratory at the University of Arizona combines engineering and medicine in the study of complex diseases and design of medical devices.
“Many of the answers to medical problems are often very similar to answers to engineering problems,” Vande Geest explained.
His Soft Tissue Biomechanics Laboratory, or STBL, applies engineering testing and analysis to human biological systems, which are similar to engineering designs, complete with bio-based pumps, valves, electrical wiring, pipes and other hardware.
The lab focuses on two research areas: improving medical device design, and identifying relationships between structure and function in soft tissues.
In one application, Vande Geest is working with Protein Genomics Inc. to grow proteins that can be molded into replacement blood vessels. Although these tubes are constructed from biological materials, the FDA treats them as medical devices, he said.
Vande Geest’s part of the project involves testing the artificial blood vessels to determine their maximum pressure ratings, strength and optimal mechanical properties for various applications.
Thwarting a Silent Killer
Another STBL project focuses on developing a patient-specific prosthesis for abdominal aneurysms that form in the aorta where it splits to feed blood to the legs. The aneurysm is a blood-filled bulge that occurs more often in men over 50 and in smokers. It is sometimes called “the silent killer,” because it generally grows without symptoms until it ruptures -- typically a fatal event.
The aneurysm is often discovered when a patient is being seen for another medical condition, said Vande Geest, who holds appointments in aerospace and mechanical engineering and in biomedical engineering.
Treatment requires either major surgery or an endovascular repair in which a stent is inserted in a leg artery and pushed into place to relieve the pressure acting on the aneurysm.
The endovascular repair is less invasive and is associated with a much quicker recovery. But the stent-grafts sometimes migrate and develop leaks, requiring additional procedures.
Vande Geest is building patient-specific devices that conform to the shape of the patient’s aorta and aneurysm, unlike current stents, which are basically tube shaped. “The hypothesis is that these patient-specific devices will limit migration and be less likely to develop leaks between the device and the aneurysm,” he explained.
The STBL devices are made from smart polymers and designed using a recently developed algorithm that automatically extracts the shape from a patient’s CT scan. The prosthesis is then built using advanced computer-aided design software and 3D printing.
“Current endovascular stent-grafts are also quite expensive, especially compared to the vascular grafts used in more traditional open surgical repair of aneurysms,” Vande Geest said. “Our hope is that this new technology will provide a more cost effective solution for endovascular repair of aneurysms.”
Research Vision
The lab’s second mission -- identifying structure-function relationships in soft tissues -- focuses on characterizing the organization of the major proteins in tissues and how those relate to function in both healthy and diseased states.
Much of this tissue characterization has focused on atherosclerosis, or stiffening, of the arteries and arterial aneurysms. Recently, Vande Geest has expanded the research to include ocular tissues, specifically in glaucoma, an eye disease marked by increased pressure within the eyeball that can lead to gradual loss of vision.
“An entire sector of the research is devoted to understanding the structural components of the eye and how those are organized, and if those components are altered during the development of glaucoma,” Vande Geest said.
“In the vascular area, understanding how components are put together in normal tissues may allow us to better construct and grow the tissues we want to replace,” he added.
In an aneurysm, the mechanical forces acting on cells weaken collagen and elastin, the primary structural components of soft tissues, Vande Geest said. The aorta’s stiffness and strength is directly related to how those two components are organized, he explained. Collagen is a connective tissue protein, which resists deformation at high loads, while elastin gives tissues their ability to bounce back into place.
In a recent study, Vande Geest showed that the abdominal aorta is mechanically and structurally different from tissue in other regions of this blood vessel, which may make it more prone to form aneurysms. He and his collaborators reached this conclusion after mechanically testing aortic tissue obtained from numerous autopsies.
In addition to test equipment that stretches tissue to determine its mechanical properties, Vande Geest has been using an intravital microscope in UA’s BIO5 building that is administered by optical microscopy expert Urs Utzinger, associate professor in the department of biomedical engineering.
Stimulating Work
Vande Geest and Utzinger use the microscope to study the organization of collagen and elastin in soft tissues without destroying them. Other microscope technologies require tissue samples that have been altered by freezing, sectioning, staining and other processes.
By studying unaltered tissues, Vande Geest’s research team gets a more accurate understanding of how the tissues function in response to mechanical stimulation. For instance, Vande Geest and Utzinger can pressurize an eyeball and study it under this microscope to investigate how glaucoma might damage nerves where they exit the eye.
In another area of research, Vande Geest is strengthening blood vessels by applying what he’s learned from analyzing how mechanical forces reorganize elastin and collagen. He and his collaborator Pak Wong, assistant professor in the department of aerospace and mechanical engineering, are using MEMs technology to create blood vessels for specific applications by stimulating tissue and encouraging cells to lay down collagen and elastin in specific structural patterns.
MEMs are microelectromechanical systems, essentially nano-scale machines that can incorporate gears, sensors, actuators and other mechanical and electrical components.
“All of our research is engineering related -- using what we know about mechanobiology to design mechanical solutions to various medical problems,” Vande Geest said.
Although much of the research, particularly in the areas of abdominal aneurysms and glaucoma, addresses specific diseases, “these technologies are not specific to one region of the aorta or to a specific disease,” Vande Geest emphasized. “Our research could apply to any tissue in which collagen and elastin are present.”